Why search for the electron EDM?
A permanent electric dipole moment (EDM) of the electron along its spin axis would violate parity (P) and time reversal (T) symmetries. Because of the CPT theorem, which states that the combined symmetry operations of parity, time, and charge conjugation must be conserved in any Lorentz-invariant theory, violation of T symmetry implies violation of CP symmetry. The CP operator effectively acts to transform
particles into anti-particles and vice versa. Therefore, violation of CP symmetry implies a difference in behavior of particles vs. antiparticles. Such a mismatch in behavior is required to explain the matter/anti-matter asymmetry of the universe. The standard model of particle physics includes CP violation, but measurements with K- and B-mesons show it is not enough to explain the cosmological matter/anti-matter asymmetry (a.k.a. the baryon asymmetry).
However, many extensions of the standard model predict new sources of CP violation that can explain the baryon asymmetry. Many of these models in turn predict a value of the electron EDM, de, large enough to be detected by our ACME experiment. By contrast, the standard model prediction for the electron EDM, de<10-39 e·cm, is far too tiny to be detected by current methods. A detectable EDM would arise from virtual emission and reabsorption of new particles that carry CP-violating forces. The smaller the EDM we can detect, the heavier the particles or the weaker the CP-violation responsible could be responsible. With any improvements in sensitivity, either evidence of new physics beyond the standard model may be discovered, or some models of new physics may be excluded.
The ACME Collaboration includes the DeMille group at the University of Chicago, the Doyle group at Harvard, and the Gabrielse group at Northwestern. ACME has set the best experimental limit on the electron EDM to date: de<1.1×10-29 e·cm.
This is sufficient to rule out many models that include new CP-violating physics at the TeV scale (and far above, in some models). With further improvements in sensitivity, the electron EDM may soon be discovered. This would unambiguously signal the existence of physics beyond the Standard Model, and set an upper bound on the energy scale where new particles must exist.
In the ACME experiment, electrons are embedded inside the polar molecule ThO. The molecules are prepared in the metastable 3Δ1 electronic state, which has two valence electrons with parallel spins. In this state, the EDM of one of these valence electrons experiences a huge effective intramolecular electric field, Eeff~ 75 GV/cm, which can produce a sizable torque even on a tiny EDM. The second valence electron serves to cancel the magnetic moment of the first, so the molecule is less sensitive to magnetic field-induced torques. ACME takes advantage of several other favorable properties of this state of ThO, including closely spaced levels of opposite parity, long spin coherence time, and suitability for use in a cryogenic molecular beam that enables high counting rates.
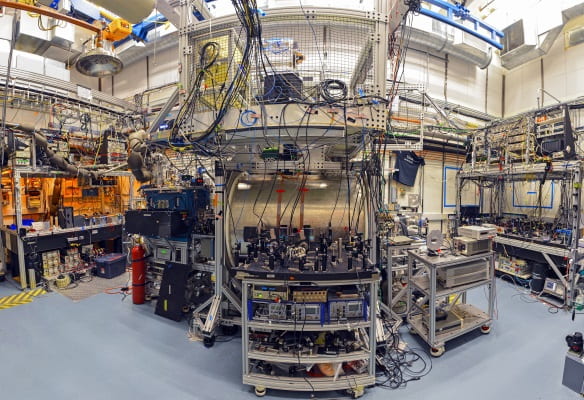
The ThO molecules are produced by ablating a ceramic target with a high-power pulsed laser. The molecules are then entrained in a flow of a cryogenic gas, producing a slow molecular beam and cooling the rotational motion of the molecules. Next, parallel electric and magnetic fields are applied to the molecules. The closely-spaced opposite parity levels repel due to the Stark effect and form two sets of states—one with the molecular axis aligned with the electric field, and the other anti-aligned. This corresponds to opposite directions of the effective intramolecular E-field. In the latest version of ACME, one molecular orientation is populated using two lasers in a coherent state preparation technique known as STIRAP. The polarization of the STIRAP lasers also aligns the electron spins. The molecular spin then precesses slowly around the magnetic field and—if the EDM is not zero—the E-field as well. We read out the final direction of the molecular spin by using a different “probe” laser, which induces molecular fluorescence only if the laser polarization aligns with the spin. The electron’s EDM will modulate the magnetic field-induced final spin angle by an amount that reverses when the experiment is repeated with molecules having the opposite molecular axis direction, which can be prepared by a small shift in the STIRAP laser frequencies. By looking at the difference of the precession angles between the two configurations, the EDM can be measured.
Our latest limit on the electron EDM represented the second generation of ACME. We are now constructing the third generation, ACME III. This involves replacing nearly all parts of the experimental apparatus aside from a few lasers, and also adding several new lasers. We are projecting another order of magnitude gain in sensitivity relative to ACME II, within the next few years. Hence, ACME III will be sensitive to new particles with mass as high as 10-300 TeV, far beyond the direct range of any high-energy collider.
Team members
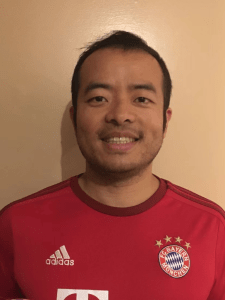
Xing Wu
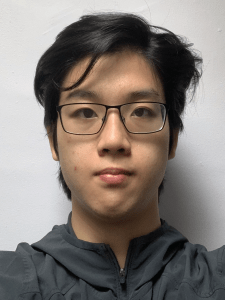
Zhen Han
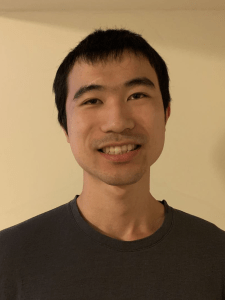